Automorphisms of the Riemann Sphere
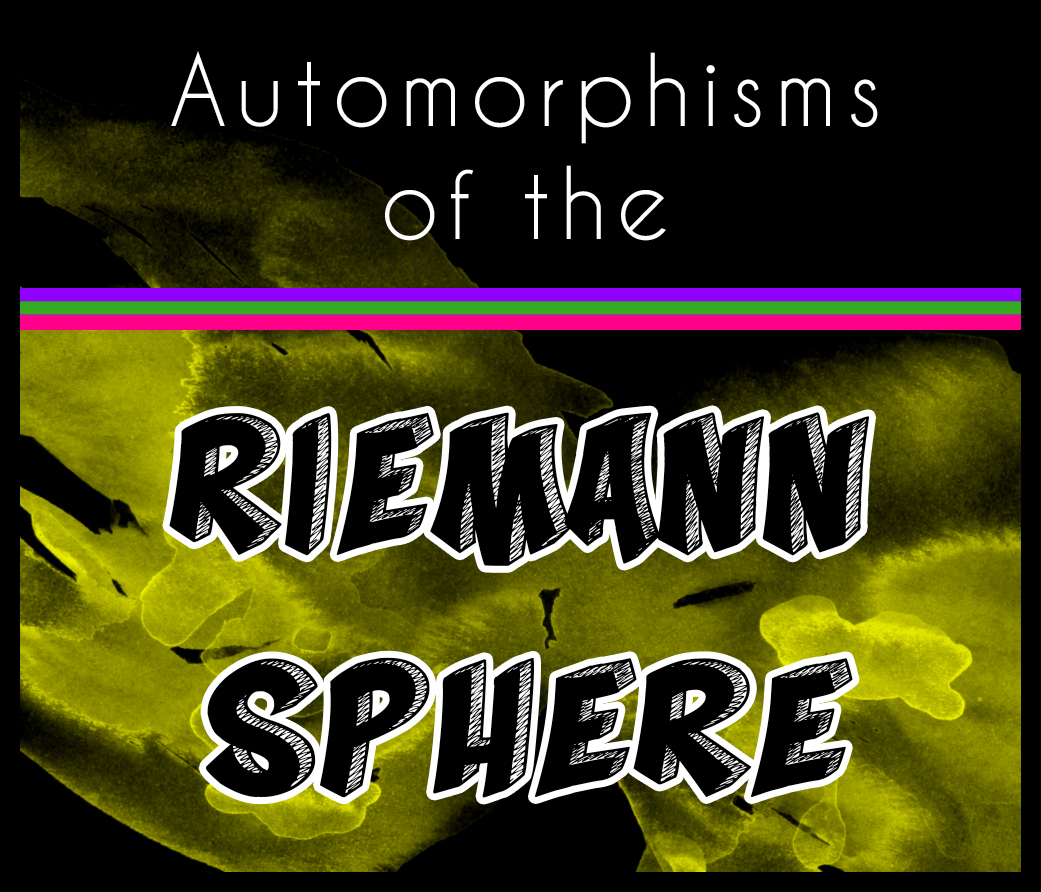
Welcome to the final post in our summer series on automorphisms of four (though, for all practical purposes, it's really three) different Riemann surfaces: the unit disc, the upper half plane, the complex plane, and the Riemann sphere. Last time, we proved that the automorphisms of the complex plane take on a certain form. Today, we'll close the series by proving a similar result about automorphisms of the Riemann sphere.
If you missed the introductory/motivational post for this series, be sure to check it out here!
Also in this series:
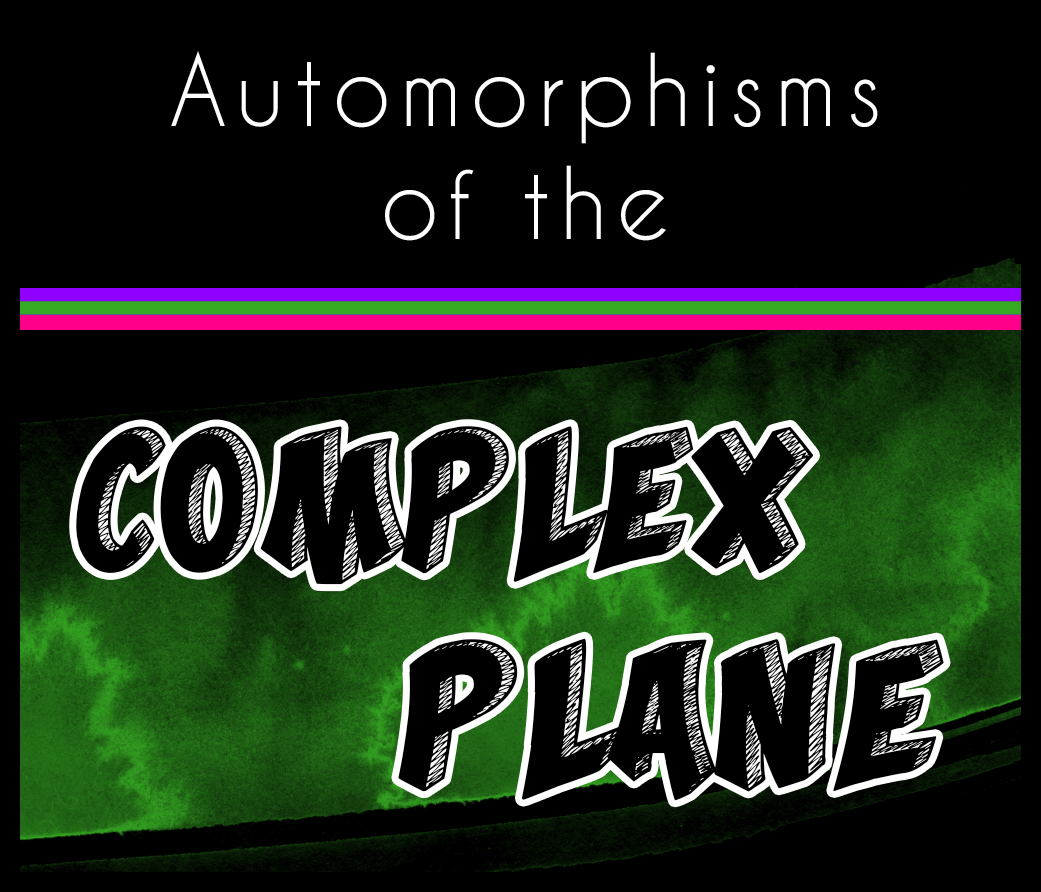
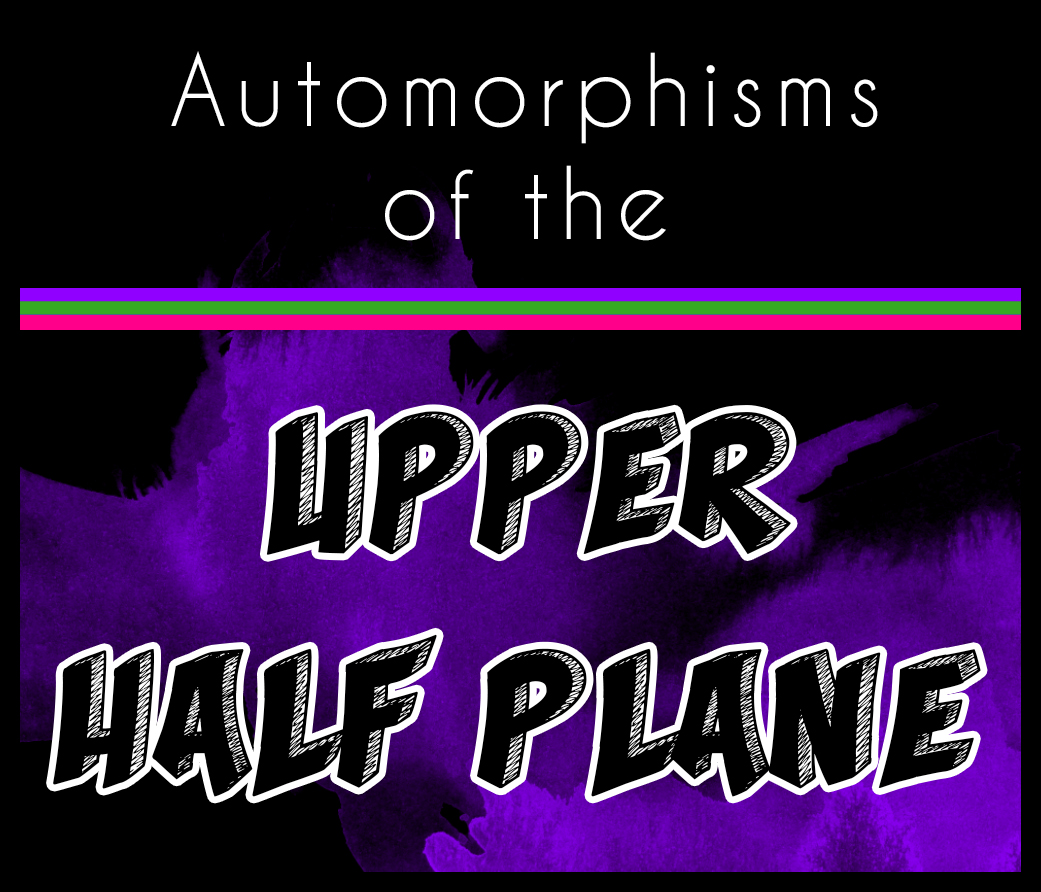

Automorphisms of the Riemann Sphere
Theorem: Every automorphism $f$ of the Riemann sphere $\hat{\mathbb{C}}$ is of the form $f(z)=\frac{az+b}{cz+d}$ where $a,b,c,d\in\mathbb{C}$ such that $ad-bc\neq 0$.
Proof. If $f(z)=\frac{az+b}{cz+d}$ for $a,b,c,d\in\mathbb{C}$ with $ad-bc\neq 0$, then $f$ is a linear fractional transformation and is thus an automorphism of $\hat{\mathbb{C}}$. The converse direction is a corollary of the following proposition.
Proposition: A function $f$ is meromorphic* in $\hat{\mathbb{C}}$ if and only if it is a rational map.
Proof. First suppose $f$ is a meromorphic function. Then $f$ has only a finite number of poles in $\hat{\mathbb{C}}$. This follows since the poles of $f$ form a discrete, closed subset of $\hat{\mathbb{C}}$. (The set is closed because its complement, the collection of points where $f$ is holomorphic, is open. To see this, note that for any point $z$ at which $f$ is holomorphic, we can find a small enough neighborhood $N$ about $z$ such that $f$ is also holomorphic on $N$.) Hence it must be finite.
So suppose the poles of $f$ are $a_1, a_2,\ldots, a_n$ and consider the Laurent expansion of $f$ at $a_1$: $$f(z)=\frac{A_{-m}}{(z-a_1)^m}+\cdots+\frac{A_{-1}}{z-a_1}+A_0 +A_1(z-a_1) + \cdots. $$ Let $p_1(z)=\frac{A_{-m}}{(z-a_1)^m}+\cdots+\frac{A_{-1}}{z-a_1}$ be the principal part of $f$ and let $f_1(z)=A_0 +A_1(z-a_1) + \cdots$ so that $f(z)=p_1(z)+f_1(z)$. Observe that $p_1$ is holomorphic for all $z\neq a_1$ and has a pole at $z=a_1$. Moreover, $f_1$ has the same number of poles as $f$ except at $z=a_1$.
Repeating this argument for each $i=1,2,\ldots, n$, we obtain the collection of principal parts $p_i(z)$ of $f$ at the pole $a_i$ . So consider the function $$f_n(z)= f(z)-p_1(z)-\cdots - p_n(z)$$ and observe that $f_n$ is holomorphic on $\mathbb{C}$ and has a pole at $\infty$. This implies that $f_n$ must be a polynomial and so $f(z)=f_n(z)+p_1(z)+\cdots p_n(z)$ is a rational function, i.e. there are polynomials $P$ and $Q$ with coefficients in $\mathbb{C}$ for which $f(z)=\frac{P(z)}{Q(z)}$.
Conversely let's assume $f=\frac{P(z)}{Q(z)}=\frac{a_nz^n+\cdots+a_0}{b_mz^m+\cdots+b_0}$ is a rational function expressed in simplest terms so that $P$ and $Q$ share no common roots. Suppose $z_0$ is a zero of $Q(z)$ of order $k$ so that $Q(z)=(z-z_0)^kQ_1(z)$ where $Q_1$ is a polynomial of degree $m-k$ satisfying $Q_1(z_0)\neq 0$. Then $$f(z)=\frac{P(z)}{(z-z_0)^kQ_1(z)}=\frac{\varphi(z)}{(z-z_0)^k}$$ where $\varphi(z)=P(z)/Q_1(z)$ is holomorphic and $\varphi(z_0)\neq 0$. It follows that $z_0$ is a pole of $f$. Hence the zeros of $Q$ are precisely the poles of $f$ (and are of the same order). Thus $f$ is meromorphic.
$\square$
Corollary: If $f$ is an automorphism of $\hat{\mathbb{C}}$ then $f$ is of the form $f(z)=\frac{az+b}{cz+d}$ where $a,b,c,d\in\mathbb{C}$ satisfy $ad-bc\neq 0$.
Proof. Suppose $f$ is an automorphism of $\hat{\mathbb{C}}$. Then $f$ must be meromorphic as $f$ must map one point to $\infty$ and thus have a pole. By the previous proposition, $f$ must be of the form $f(z)=\frac{P(z)}{Q(z)}$ for polynomials $P$ and $Q$. But by assumption $f$ is injective and so $P$ and $Q$ must be linear in $z$. In other words $f(z)=\frac{az+b}{cz+d}$ for $a,b,c,d\in\mathbb{C}$. (And a quick check shows that the condition $ad-bc\neq 0$ is needed to guarantee that $f^{-1}$ exists.) This completes the proof of the Theorem above.
$\square$

*that is, $f$ is holomorphic on all of $\hat{\mathbb{C}}$ except for a set of isolated points, namely the poles of $f$.